Summary and Commentary on the Physical Review Letter: “Sound-based analogue of cavity quantum electrodynamics in silicon” by O. Soykal, R. Ruskov, and C. Tahan. (Posted to the arxiv on June 8, 2011.)
Read the accompanying APS Focus article: Vibrations and electrons team up to form new quantum entity
We now have the ability to construct increasingly complex artificial quantum systems, with profound implications for science and technology. This work explores the possibility of a new, man-made quantum object: a hybridization of a localized, long-lived phonon (a quantum of sound) and a matter excitation. That this is possible is not obvious. Analogous to the case of cavity-QED where a photon can strongly couple with a matter excitation and become a polariton (a half-light, half-matter quasiparticle), here a phonon in a crystal plays the part of the photon. We call this half-sound, half-matter object a “phoniton”. Our results show that phoniton-based devices are possible in silicon. The phoniton can augment existing tools to manipulate quantized vibrations in nanoscale mechanical systems, help us further understand the nature of sound and heat, and serve as a base component in new macroscopic artificial quantum systems.
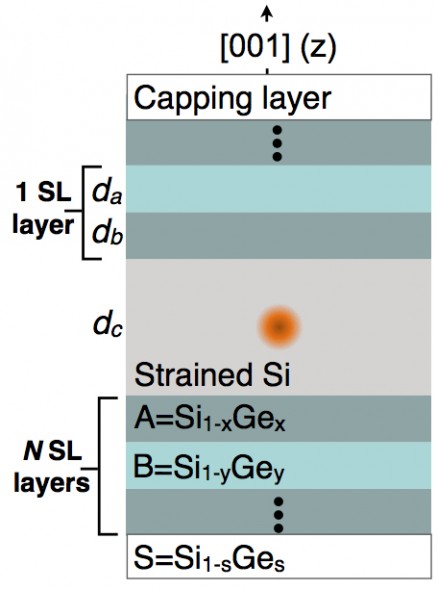
A system where a phonon-analog of cavity-QED can be realized. Here a donor atom such as Phosphorous is placed in a SiGe acoustic distributed Bragg reflector (DBR) cavity. Other two-level systems and materials are possible. Very strong phonon-TLS coupling can be achieved due to the large deformation potential typical of semiconductor crystals.
A number of conditions must be met to realize a cavity-phoniton. First and foremost, the two-level system (TLS) must be driven solely by phonons. Second, the excited state should emit into the preferred phonon mode of the cavity and not into other phonon or photon modes (which would be considered loss). Third, a phonon cavity must isolate a single phonon mode (of the appropriate type and frequency) and trap it for sufficient time to interact with the impurity transition. Fourth, the phonon-TLS coupling strength must be large relative to leakage from the cavity, disintegration of the phonon into other modes, and phonon-phonon or phonon-impurity scattering; strong coupling in the sense of cavity-QED is achieved. We have showed theoretically that these conditions can be met in a realizable, silicon-based device.
Although a proposal for the quantum amplification of sound (a phonon maser) appeared as early as the 60s, the pursuit of classical and quantum phonon technology has accelerated recently as our ability to nanofabricate and manipulate quantum systems has improved. Phonons are slow compared to light, a property that is already used, e.g., in signal processing. Their smaller wavelengths are potentially relevant for nanoscale imaging and devices. Phonon analogues of lasers have been proposed and demonstrated. In quantum information processing, phonons can be decoherence pathways as well as tools for coupling quantum systems, for example, in ion traps or solid-state qubits, and have even been considered for simulation of many-body dynamics.
These components can be added to phononics as a useful tool much like in the case of photonics (memory, filtering, etc.). The system can also be extended to many-phoniton systems which can exhibit novel quantum many-body behavior – which may be the true potential of systems like these for device applications. For example, “solid-sound” systems in analogy with coupled cavity-QED solid-light systems could demonstrate Mott insulator like states of phonons in coupled phoniton cavities. Finally, cavity/qubit geometries such as these may also be relevant for quantum computing (QC) applications: to mediate interactions between distant qubits or inhibit decoherence in structures not dramatically different from those used today.
Visualization of the phoniton. The calculated phonon displacement field in a silicon micropillar cavity is shown with the white arrows; the wave function of the donor electron it interacts with is shown as the color probability distribution.